Scientist works on artificial tissue development
Maria Helguera contributes ultrasound techniques, image processing to NIH-funded project
The beginnings of artificial vascular networks created with ultrasound waves. The frequency and intensity of the waves organize the cells into position.
Circulating oxygen-rich blood through artificial organs and tissues is a bioengineering conundrum without an easy answer. The problem is in the plumbing. Biomedical teams around the world are working on different solutions to the problem of creating a synthetic vascular system.
Scientists at Rochester Institute of Technology and the University of Rochester are looking to ultrasound technology to create tiny blood vessels needed to nourish organs and tissues grown for reconstructive and surgical applications.
Developing complex vascular systems with high-frequency ultrasound waves is the goal of RIT imaging scientist Maria Helguera ’99 (Ph.D., imaging science), and UR’s Diane Dalecki, professor of biomedical engineering, and Denise Hocking, associate professor of pharmacology and physiology. The UR-led project is funded by the National Institute of Biomedical Imaging and Bioengineering, part of the National Institutes of Health.
Helguera provides the team an expertise in ultrasound imaging and image processing through high-frequency ultrasound techniques and quantitative analysis of microscopy images of the tissue samples.
“We can use ultrasound in every stage of the process while creating artificial tissue,” said Helguera, an associate professor at RIT’s Chester F. Carlson Center for Imaging Science. “Ultrasound is a clean way of doing things in the sense that we are not delivering any harmful radiation. We can manipulate the cells and image them without hurting the samples.”
Ultrasound standing wave fields are generated in a tissue-culture plate containing endothelial cells embedded in collagen. Endothelial cells form the insides of blood vessels. Pressure from ultrasound standing wave fields nudges the cells into predetermined positions. The frequency and intensity of the waves organize the cells and control the density and spacing of cell bands. Samples are then polymerized in an incubator and locked into place. Their close positioning encourages cells to signal to each other and sprout three-dimensional blood vessels.
Tissue constructs are visualized with multiphoton microscopy, a technique that captures specimens in three-dimensional sections.
“Distinct and interesting formations can be seen depending on the frequency and intensity of the ultrasound stationary wave fields,” Helguera said. “We decided to quantitatively analyze these three-dimensional data sets to extract parameters characteristic of each exposure regime.”
Imaging science Ph.D. student Mohammed Yousefhussien developed an image-processing tool for evaluating the structures of the blood sprouts. Third-year imaging science student Amy Becker is modifying the tool to capture details that will help manipulate the sprouts’ growth. Determining the preferred direction in which the vessels branch outward will lead to networks resembling the vascular system within an organ.
“The goal is to design a quantitative protocol that will allow us to create a more complicated structure that is closer to a real system,” Helguera said.
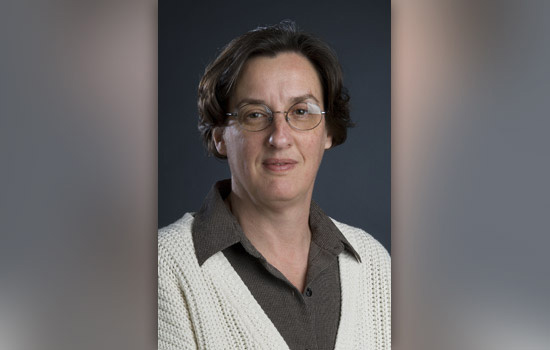